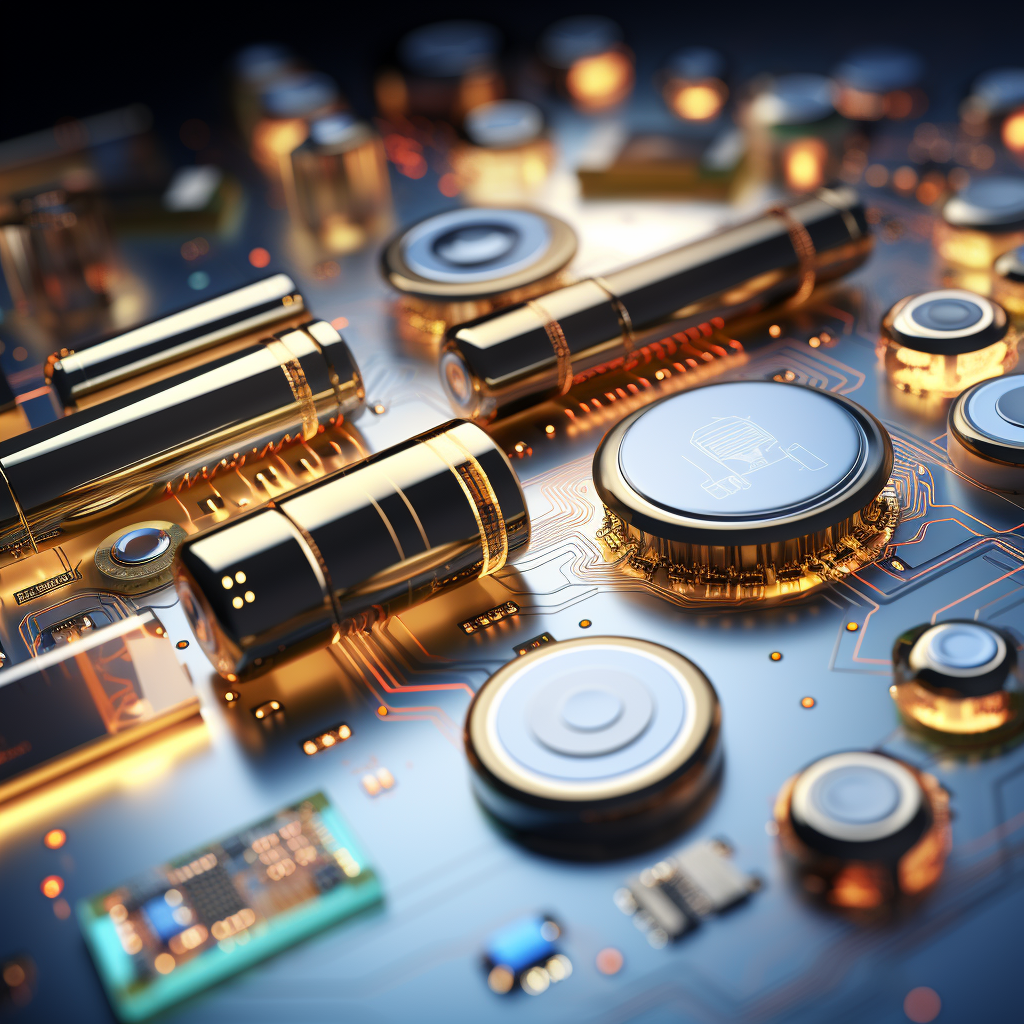
Unlocking the Potential of Lithium-Ion Battery Chemistries
- 03 Sep, 2023
The advent of lithium-ion batteries has been nothing short of transformative. Since their commercial introduction in the early 1990s, these energy storage systems have found application in everything from portable electronics like smartphones and laptops to electric vehicles and renewable energy systems. But what makes lithium-ion batteries so versatile and powerful? The answer lies in their chemistry.
Battery chemistry is not a one-size-fits-all scenario. Different chemistries offer varied benefits, drawbacks, and suitability for particular applications. Understanding these chemistries can help us make more informed decisions—whether it's choosing a smartphone that lasts all day or an electric vehicle that can go the distance.
In this article, we will delve deep into the world of lithium-ion battery chemistries. We'll explore the science that makes them work, compare different types of chemistries, weigh their pros and cons, and evaluate their applications. We'll also touch upon safety considerations and the future of lithium-ion technologies. Our objective is to provide a comprehensive guide that serves both technical and non-technical audiences, adhering to the principles of expertise, authority, and trustworthiness.
Whether you're a consumer looking to understand the gadget you rely on daily, or an engineer aiming to specify the right kind of battery for a particular application, this article aims to be your definitive guide to the complex world of lithium-ion battery chemistries.
A Brief History of Lithium-ion Batteries
The tale of lithium-ion batteries is one of innovation and technological evolution. Before delving into the complexities of their chemistries, it's essential to understand how they came to be a central element in our lives.
The Pioneering Phase
The lithium-ion battery journey starts with early research in the 1970s. Pioneering work by chemists like John Goodenough laid the foundation for what would become a transformative technology. Goodenough's invention of lithium-cobalt-oxide cathodes in 1980 was a game-changing development that opened the door for rechargeable lithium-ion batteries.
Commercial Breakthrough
The first commercial lithium-ion battery was introduced by Sony in 1991. Utilizing lithium-cobalt-oxide, this battery was lighter and offered higher energy density compared to previous nickel-cadmium (NiCd) and nickel-metal-hydride (NiMH) batteries. The release marked the beginning of a new era in portable electronics, eventually making possible the gadgets we can't live without today.
Diversification and Optimization
Initially, lithium-cobalt-oxide batteries were the norm, but they had limitations, such as sensitivity to high temperatures and a risk of thermal runaway, which could result in fires. Researchers started exploring other chemistries like lithium-manganese-oxide and lithium-iron-phosphate, which offered more stability. Over the years, the chemistries have been fine-tuned for specific applications, from electric vehicles to medical devices.
The Rise of Electric Vehicles
The 2010s saw an explosive growth in the use of lithium-ion batteries for electric vehicles (EVs). Companies like Tesla played a significant role in advancing battery technology and manufacturing. In EVs, lithium-ion batteries with different chemistries, such as lithium-nickel-manganese-cobalt-oxide (NMC), started gaining prominence due to their balance between high energy density and safety.
Sustainable and Safe Technologies
With increasing awareness of climate change and the need for sustainable technologies, lithium-ion batteries have gained attention as a renewable energy storage solution. Additionally, safety innovations like advanced Battery Management Systems (BMS) have been developed to mitigate risks associated with battery use.
A Glimpse into the Future
As of 2022, research into next-generation lithium-ion batteries, like solid-state batteries, continues at a rapid pace. The ultimate goal is to achieve higher energy density, quicker charging, and better safety profiles.
The Science Behind Lithium-ion Batteries
Understanding lithium-ion batteries necessitates a basic grasp of the science that fuels them. Though the topic can become rather complex, we will aim to distill it into essential elements that make these batteries so versatile and efficient. This chapter is your gateway to appreciating why different chemistries matter.
How Does a Lithium-ion Battery Work?
A typical lithium-ion battery consists of three main components: the anode (usually made from graphite), the cathode (where the specific chemistry comes into play), and the electrolyte (often a lithium salt in an organic solvent). When the battery discharges, lithium ions move from the anode to the cathode through the electrolyte, releasing energy. During charging, the ions move in the opposite direction.
Energy Density
Energy density is a critical factor that dictates how much power a battery can hold. It is usually measured in watt-hours per kilogram (Wh/kg). Lithium-ion batteries tend to have higher energy densities compared to older technologies like NiCd and NiMH, making them ideal for applications that require compact and lightweight power sources.
Voltage and Capacity
Voltage determines the potential difference between the anode and cathode, affecting how much energy can be extracted from the battery. Capacity, typically measured in ampere-hours (Ah), dictates how long the battery can deliver its rated voltage. Different chemistries can tweak these parameters to suit specific applications.
Cycle Life
Cycle life is the number of complete charge and discharge cycles a battery can undergo before its capacity significantly diminishes. Some lithium-ion chemistries offer longer cycle lives than others, making them more suitable for applications like electric vehicles, where frequent charging is required.
Thermal Stability
Thermal stability is a crucial safety aspect. Some lithium-ion chemistries are more stable than others at high temperatures, reducing the risk of thermal runaway—a chain reaction that can lead to overheating and, in extreme cases, fire or explosion.
Charging Speed
The ability to rapidly charge and discharge is another factor influenced by the battery's chemistry. Some applications, like fast-charging EV stations, require batteries that can handle quick energy transfer without compromising longevity or safety.
Understanding these basic principles and parameters allows us to appreciate the significant role that different lithium-ion chemistries play in optimizing batteries for various applications—from your smartphone that needs to last all day on a single charge, to an electric car that requires fast charging and a high energy density for long-range travel.
Types of Lithium-ion Battery Chemistries
The lithium-ion landscape is dotted with a variety of chemistries, each offering a unique blend of attributes. This chapter aims to explore these in-depth, shedding light on their characteristics, advantages, and limitations. By doing so, we can better understand how these chemistries align with different applications.
Lithium Cobalt Oxide (LiCoO2)
- Characteristics: High energy density, relatively low thermal stability
- Applications: Smartphones, laptops, cameras
- Pros: Compact and lightweight
- Cons: Expensive and sensitive to high temperatures
Lithium Cobalt Oxide was the first commercial lithium-ion chemistry and remains the most commonly used for portable electronics like smartphones and laptops. Its high energy density allows for compact designs but at the cost of thermal sensitivity, which can pose safety concerns.
Lithium Manganese Oxide (LiMn2O4)
- Characteristics: Lower energy density, better thermal stability
- Applications: Medical devices, power tools
- Pros: Safer and more stable at high temperatures
- Cons: Lower capacity and shorter cycle life
For applications that prioritize safety over energy density, Lithium Manganese Oxide provides a viable option. This chemistry offers good thermal stability, making it suitable for medical devices and power tools, where safety is paramount.
Lithium Iron Phosphate (LiFePO4)
- Characteristics: Lower energy density, high thermal stability, long cycle life
- Applications: Electric vehicles, renewable energy storage
- Pros: Extremely safe and durable
- Cons: Bulkier and less energy-dense
Lithium Iron Phosphate excels in safety and durability. Its long cycle life and stability at high temperatures make it an ideal choice for electric vehicles and large-scale energy storage systems where safety and longevity are crucial.
- Lithium Nickel Manganese Cobalt Oxide (LiNiMnCoO2 or NMC)
- Characteristics: High energy density, balanced thermal stability
- Applications: Electric vehicles, portable power banks
- Pros: Good balance between energy density and safety
- Cons: Moderate cost and complexity in manufacturing
This is a 'best-of-both-worlds' chemistry that combines high energy density with decent thermal stability. Its balanced set of attributes makes it a popular choice for electric vehicles and high-capacity portable chargers.
Understanding these chemistries helps to demystify the factors that go into selecting a battery for a specific need. Whether it's a lightweight battery for your smartphone or a robust energy storage solution for an off-grid solar installation, the chemistry plays a crucial role in defining its performance metrics.
Advantages and Disadvantages of Each Chemistry
When selecting a lithium-ion battery for a specific application, one must weigh various factors such as energy density, safety, longevity, and cost. Each lithium-ion chemistry presents a unique blend of advantages and disadvantages, which we'll explore in depth in this chapter.
Lithium Cobalt Oxide (LiCoO2)
Advantages
- High Energy Density: Ideal for portable electronics that require long-lasting power in a compact form.
- Mature Technology: Well-understood and widely available due to its long history.
Disadvantages
- Cost: Generally more expensive due to the high cost of cobalt.
- Safety Concerns: Prone to thermal runaway, especially when damaged or improperly handled.
Lithium Manganese Oxide (LiMn2O4)
Advantages
- Safety: Offers better thermal stability, reducing safety risks.
- Cost-Effective: Manganese is more abundant and less expensive than cobalt.
Disadvantages
- Lower Energy Density: Not suitable for applications that require compact, high-energy storage.
- Shorter Cycle Life: Tends to degrade faster, reducing its overall lifespan.
Lithium Iron Phosphate (LiFePO4)
Advantages
- Safety: Exceptional thermal stability makes it one of the safest lithium-ion chemistries.
- Long Cycle Life: Highly durable, offering longer lifespan especially for high-drain applications.
Disadvantages
- Size and Weight: The lower energy density results in bulkier batteries.
- Lower Voltage: Typically has a lower nominal voltage, which may not be suitable for certain applications.
Lithium Nickel Manganese Cobalt Oxide (NMC)
Advantages
- Balanced Properties: Offers a good mix of high energy density and thermal stability.
- Versatility: Suitable for a wide range of applications, from EVs to power banks.
Disadvantages
- Cost: The blend of materials makes it moderately expensive.
- Complex Manufacturing: Requires a more complex synthesis process, which can impact scalability.
Understanding the pros and cons of each lithium-ion chemistry allows users and manufacturers to make informed decisions tailored to specific needs. Whether the priority is longevity, safety, or energy density, there's a chemistry suited for that application.
Applications: Where Each Chemistry Excels
Given the variety of lithium-ion battery chemistries available, it's imperative to match the right chemistry with its ideal application. Whether it's the need for high energy density in smartphones or robust safety measures in electric vehicles, different chemistries offer tailored solutions. This chapter will delve into real-world applications that most benefit from each type of lithium-ion chemistry.
Lithium Cobalt Oxide (LiCoO2): The Powerhouse for Portable Electronics
This chemistry is the go-to choice for most consumer electronics like smartphones, laptops, and cameras. Its high energy density allows for slim and lightweight designs, making it the preferred option for devices that prioritize portability.
Lithium Manganese Oxide (LiMn2O4): The Safety-First Choice
LiMn2O4 finds its calling in applications that demand a higher level of safety, like medical devices and power tools. For instance, automated external defibrillators (AEDs) often use this chemistry due to its higher thermal stability.
Lithium Iron Phosphate (LiFePO4): The Long Runner
When it comes to large-scale, long-term applications like electric vehicle powertrains and solar energy storage systems, LiFePO4 is a standout choice. Its long cycle life and robust safety features make it ideal for high-drain, heavy-duty applications.
Lithium Nickel Manganese Cobalt Oxide (NMC): The Balanced Performer
Electric vehicles and high-capacity power banks often use NMC batteries. The chemistry offers a balance between high energy density and thermal stability, making it versatile for a range of applications that require both power and safety.
Special Mention: Emerging Applications
Technologies like drones and wearable devices often require a blend of properties from different chemistries, such as both high energy density and thermal stability. In such cases, custom lithium-ion solutions or hybrid chemistries are sometimes developed to meet these specialized needs.
By matching the optimal chemistry to each application, manufacturers can ensure not only better performance but also enhanced safety and durability. This targeted approach has been key in realizing the full potential of lithium-ion technology, driving innovations across multiple industries.
Conclusion and Future Outlook
We've traversed the intricate landscape of lithium-ion batteries, demystifying their core principles, exploring the various chemistries, and delving into their applications. As we've seen, each chemistry offers its unique set of advantages and disadvantages, making them suitable for specific uses. Whether it's the high energy density of Lithium Cobalt Oxide for smartphones or the unparalleled safety of Lithium Iron Phosphate for electric vehicles, understanding these nuances is critical for making informed decisions.
Future Outlook
The realm of lithium-ion batteries is not static; it's an evolving field ripe for innovation. Research is underway to overcome current limitations, such as safety concerns and resource scarcity, through various means:
- Resource Sustainability: The hunt for alternative, more abundant materials to replace costly and rare elements like cobalt is gaining momentum.
- Improving Safety: Research focuses on enhancing thermal stability and developing safer electrolytes to minimize risks associated with lithium-ion batteries.
- Boosting Performance: Efforts to improve energy density and cycle life are constant, aiming to make lithium-ion batteries even more efficient and long-lasting.
- Recycling and Repurposing: As the adoption of lithium-ion technology grows, so does the need for effective recycling and waste management strategies.
- Emerging Technologies: Solid-state lithium-ion batteries and lithium-air batteries are among the technologies that could redefine our understanding of portable energy storage.
In this ever-evolving landscape, staying updated on the latest advancements is crucial for both consumers and manufacturers. As we continue to push the boundaries of what's possible with lithium-ion technology, the key takeaway remains: the right chemistry can significantly influence a battery's performance, safety, and suitability for any given application.
By understanding the complex interplay of these factors, we are better equipped to harness the full power of lithium-ion batteries, driving advancements that benefit not just individual consumers, but society at large.
References
Goodenough, John B.; Park, Kyu-Sung (2013). "The Li-Ion Rechargeable Battery: A Perspective" (PDF). Journal of the American Chemical Society. https://pubs.acs.org/doi/abs/10.1021/ja3091438
Tarascon, J. M.; Armand, M. (2001). "Issues and challenges facing rechargeable lithium batteries". Nature. https://www.nature.com/articles/35104644
"Types of Lithium-ion Batteries". Battery University. https://batteryuniversity.com/article/bu-205-types-of-lithium-ion